The landscape within the Southwest Climate Adaptation Science Center (SW CASC) boundary is extremely varied. It ranges from the Sonoran and Mojave Deserts to the California Coast; from the Sierra Nevada Mountains to the Colorado Plateau and Southern Rockies; from the Great Basin to the Madrean Sky Islands. The topographic extremes within these provinces drive an immense range of variability in climate, ecosystems, and hydrologic systems. The Southwest region includes the highest (Mount Whitney, 14,494 ft) and lowest (Death Valley, -282 ft) points in the conterminous United States. Such varied topography strongly influences temperature, precipitation, evaporation, soils, and other variables that affect hydrologic and ecologic conditions. Precipitation in the area ranges from about 250 cm annually in parts of northern California to less than 8 cm annually in Death Valley, with snowfall dominating in some areas and virtually absent in others. Surface-water hydrology includes large snowmelt-dominated rivers, such as the Colorado and Sacramento-San Joaquin Rivers, base-flow supplied perennial streams, and extensive ephemeral systems. The region’s ecosystems reflect the diversity of topography and climate, and include deserts, woodlands, chaparral, tundra, marine systems, coastal wetlands, and forests ranging from coastal to montane to subalpine. The Southwest also supports extensive human land uses, and includes some of the nation’s most productive agricultural regions and largest urban areas.
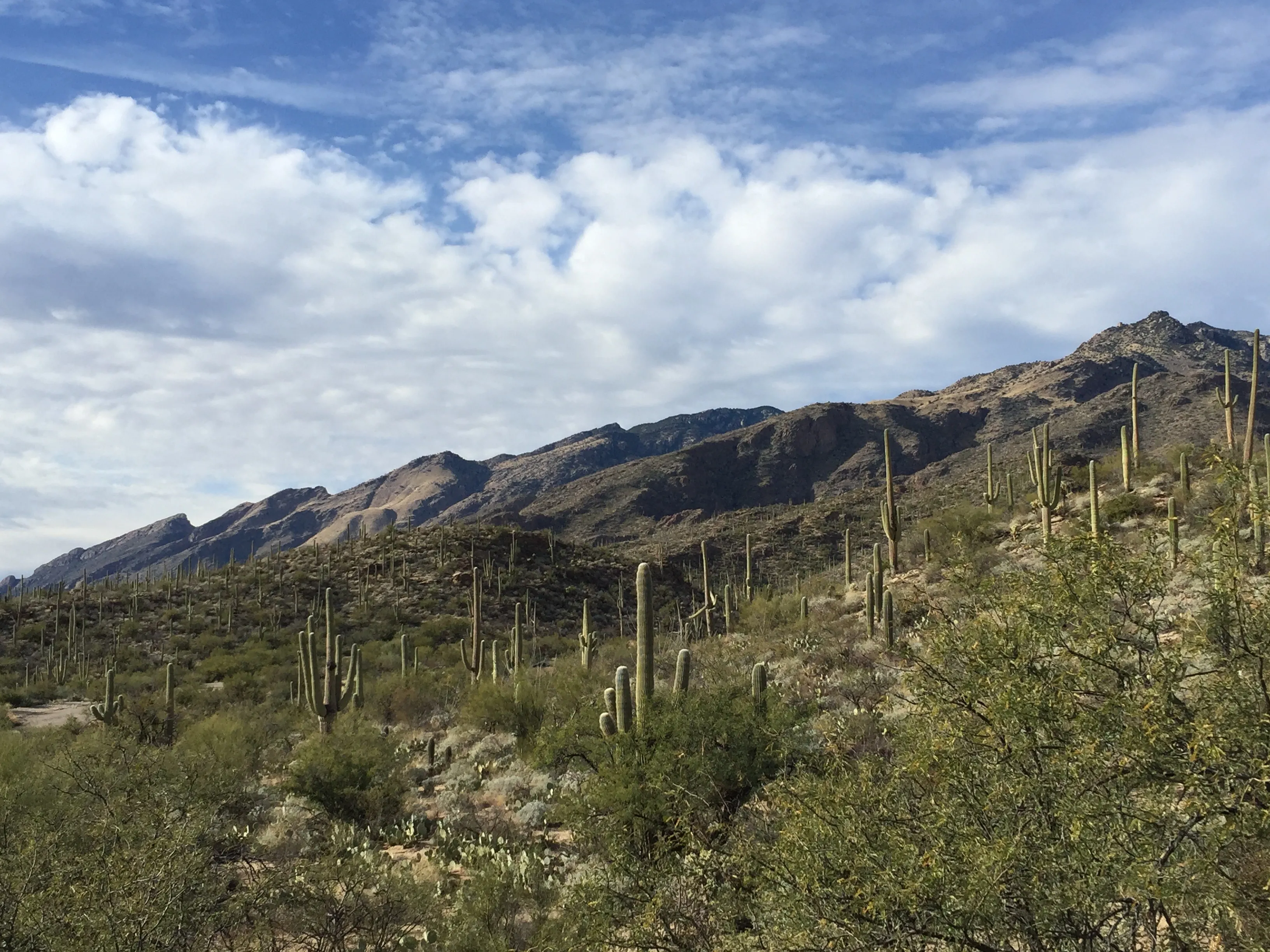
Christine Schirmer
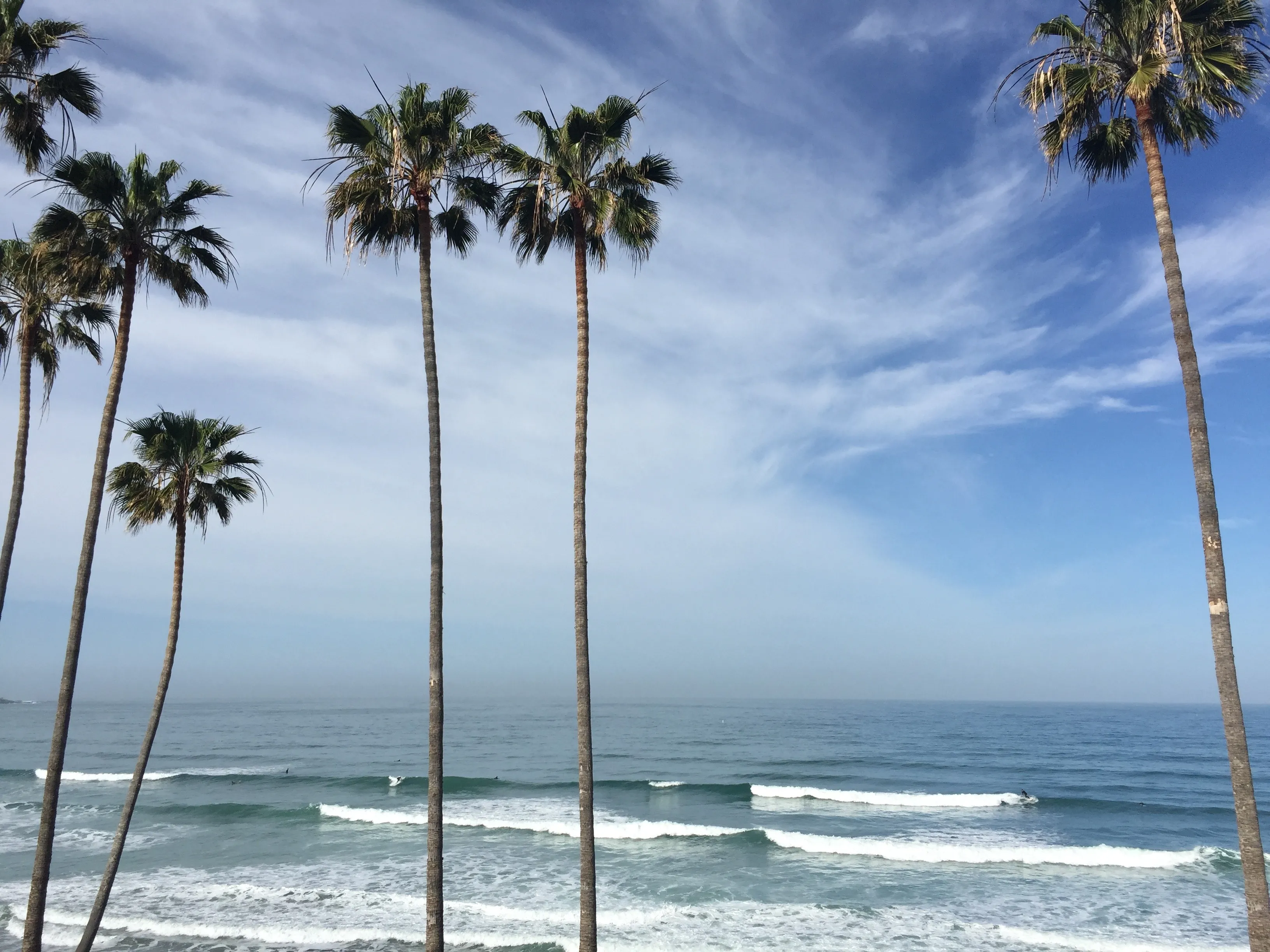
Christine Schirmer
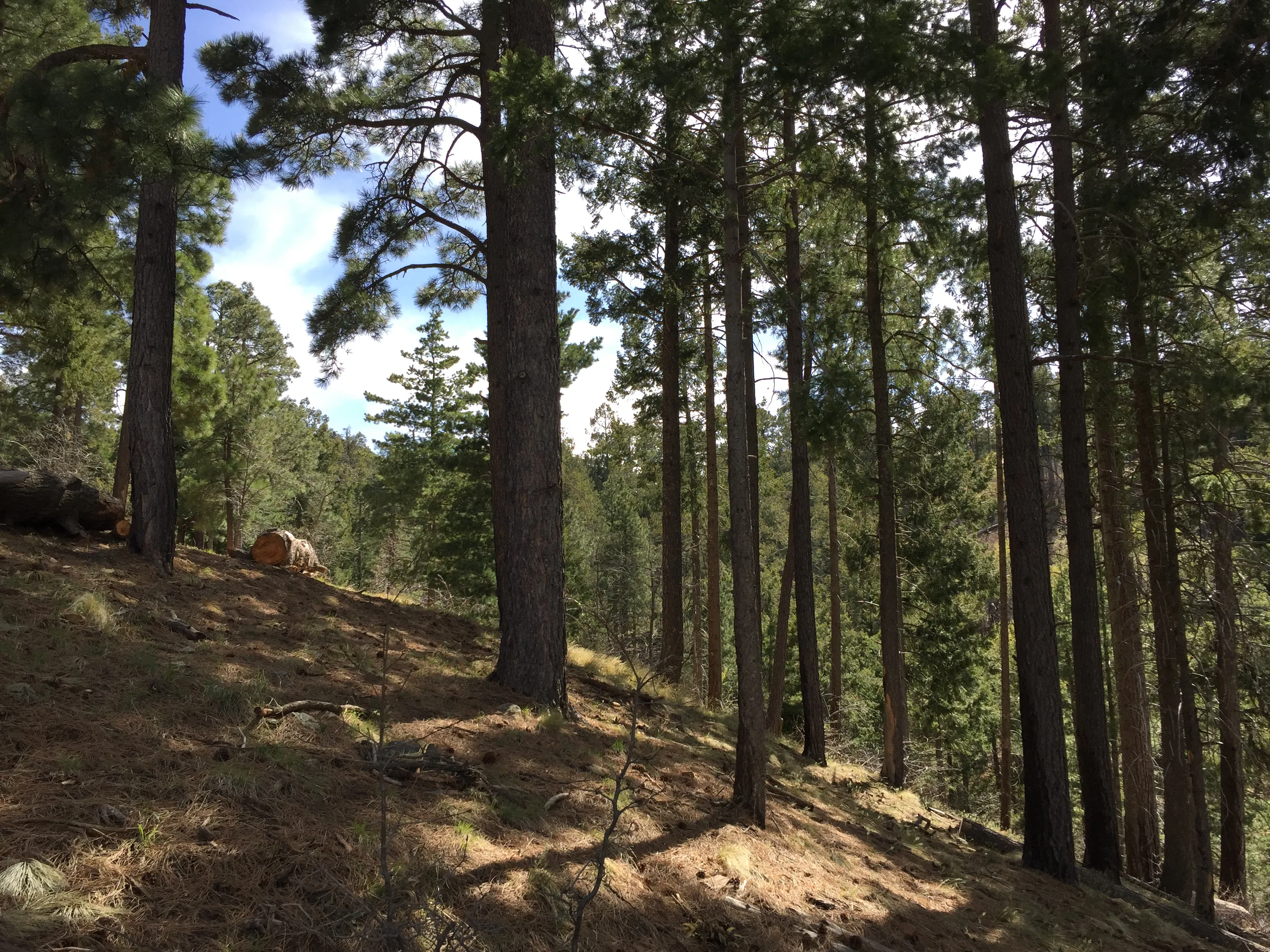
Christine Schirmer
Non-climate stresses on southwestern ecosystems and hydrologic systems increased dramatically through the 20th century as human populations expanded and human activities (for example, grazing, mining, irrigation, and urbanization) intensified. Population in the region is projected to increase through the 21st century. Land-use and management practices will change as population increases. Similarly, anthropogenic water demand has increased as population has increased in many areas that are already water-limited.
Natural climate variability combined with on-going and increasing levels of anthropogenic climate change both pose serious challenges for the Southwest and Southwestern resource managers. The Southwest is naturally the most drought-prone region of the U.S., and since 1999 has been experiencing the longest and hottest drought on record for the U.S. (Woodhouse & Overpeck, 1998; Cook et al., 2015; Udall & Overpeck, 2017). This drought has led to major reductions in runoff and river flow (e.g., flows of the largest river of the Southwest, the Colorado River, have averaged 19% below the 20th century average since 2000; Udall & Overpeck, 2017). If the drought continues, emergency measures, including water rationing, are anticipated in the lower Colorado River Basin by 2017 or 2018.
The drought, accompanied by unusually high temperatures, is also responsible for the most widespread mortality of trees in the U.S. (e.g., in the Four-corners region, as well as California; Breshears et al., 2005; Van Mantgem et al., 2009; Williams et al., 2010). The record long, and record hot drought, has also contributed to widespread tree mortality due to insect and pathogen outbreaks, as well as unusually severe large wildfires (Williams et al., 2010; Williams et al., 2013). Most recently, it is driving direct physiological mortality of trees in the Sierra Nevada region.
It is now well established that the unprecedented Southwest drought has been exacerbated by anthropogenic warming, but it is also well documented that droughts lasting decades regularly plague the Southwest, with the longest of these “megadroughts” yet documented in the last 2000 years, being 50+ years (Routson et al., 2011). Projected 21st-century warming will increase the odds of a multidecadal megadrought in the Southwest from less than 15% to over 90% if there is no increase in mean precipitation; even if modest precipitation increases do occur, the risk will still exceed 70% (Ault et al., 2014).
Projections for future climate and water resources in the area are largely consistent with trends observed in recent decades. Temperatures have been rising across the region, and are projected to to continue increasing, with higher maxima and minima and higher numbers of high-temperature days annually (Garfin et al., 2013). Future water availability for people and ecosystems will change in less predictable ways. Although some climate models suggest increases in precipitation, others do not; moreover, there has been no trend toward greater precipitation (Udall & Overpeck, 2017). Hydrological models project more frequent drought with winter snowpack likely to decline, but changes in precipitation amounts and intensity will vary across the landscape. Additionally, changes in the seasonality of precipitation are probable and trends toward earlier melting are likely to continue. Finally, increasing temperatures are likely to offset even the most optimistic scenarios for precipitation owing to non-linear effects on vapor-pressure deficit. To sum up, the region will become increasingly water-stressed under all plausible precipitation scenarios under projected future warming.
Ecological responses to climate variability and change are complex and diverse, and will likely extend well beyond the highly visible ecological responses described above. Many species are adjusting their elevational ranges upward (e.g., Parmesan, 2006; Brusca et al., 2013), but patterns are often complex, with some species shifting downward or staying in place (Loarie et al., 2008). Phenological patterns are also shifting across the region, and aquatic systems are challenged as well, with the very real possibility of species extinction given sufficient climate warming and drying (Overpeck & Bonar, 2017). Rapid, landscape-scale mortality of dominant trees and shrubs (wildfires, pests, physiological stress) is resetting successional clocks to zero, and may herald region-wide ecosystem transformations in the near future, with large consequences for biodiversity and ecosystem services.
In addition to on-going warming, sea-level rise is the other climate-related change that is already occurring and sure to continue in the future (Garfin et al., 2013; Church et al., 2013). Rising sea levels resulting from global climate change will affect these systems in many ways, including inundation of low-lying areas, erosion of coastlines, and increased salinity in estuaries. These effects will compound when sea-level rise is superimposed on natural climate cycles, storms, and tides, as well as the impacts of ocean acidification and other biogeochemical changes (Pörtner et al., 2014).
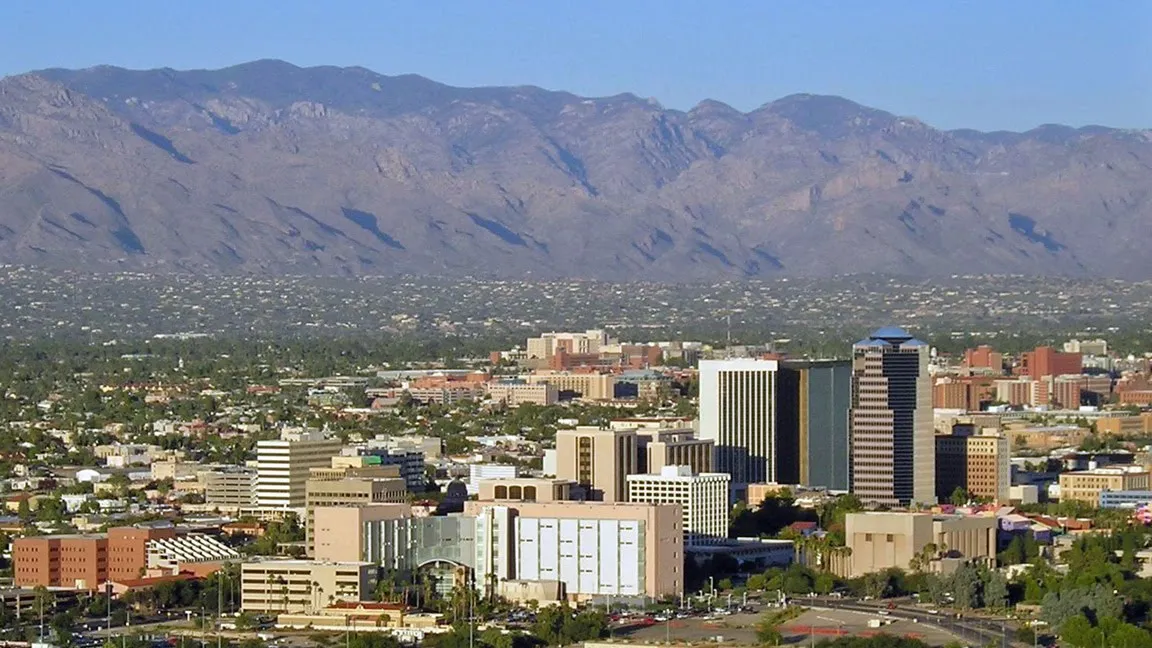
Human populations of the Southwest rely on the physical and biological resources of the region for water, food, and recreation. The climate-induced changes to the quality and availability of these resources described underscores the importance of effectively understanding the needs of resource managers and delivering the best science available to them. Landscapes in the Southwest are inextricably linked to the identity and economic well-being of individuals, communities, and the region as a whole, underscoring the importance of the CASC enterprise that connects the informational needs of resource managers with the best scientific capacity available.
Ault, T. R., Cole, J. E., Overpeck, J. T., Pederson, G. T., & Meko, D. M. (2014). Assessing the risk of persistent drought using climate model simulations and paleoclimate data. Journal of Climate, 27(20), 7529-7549.
Breshears, D. D., Cobb, N. S., Rich, P. M., Price, K. P., Allen, C. D., Balice, R. G., Romme, W. H., Kastens, J. H., Floyd, M. L., & Belnap, J. (2005). Regional vegetation die-off in response to global-change-type drought. Proceedings of the National Academy of Sciences of the United States of America, 102(42), 15144-15148.
Brusca, R. C., Wiens, J. F., Meyer, W. M., Eble, J., Franklin, K., Overpeck, J. T., & Moore, W. (2013). Dramatic response to climate change in the Southwest: Robert Whittaker's 1963 Arizona Mountain plant transect revisited. Ecology and Evolution, 3(10), 3307-3319.
Church, J. A., Clark, P. U., Cazenave, A., Gregory, J. M., Jevrejeva, S., Levermann, A., Merrifield, M. A., Milne, G. A., Nerem, R. S., & Nunn, P. D. (2013). Sea level change. Climate Change 2013: The Physical Science Basis. Contribution of Working Group I to the Fifth Assessment Report of the Intergovernmental Panel on Climate Change.
Cook, B. I., Ault, T. R., & Smerdon, J. E. (2015). Unprecedented 21st century drought risk in the American Southwest and Central Plains. Science Advances, 1(1), e1400082.
Garfin, G., Jardine, A., Merideth, R., Black, M., & LeRoy, S., eds. (2013). Assessment of Climate Change in the Southwest United States: A report prepared for the National Climate Assessment. Washington DC: Island Press.
Loarie, S. R., Carter, B. E., Hayhoe, K., McMahon, S., Moe, R., Knight, C. A., & Ackerly, D. D. (2008). Climate change and the future of California's endemic flora. PloS one, 3(6), e2502.
Overpeck, J., & Bonar, S. A. (2017). Southwestern fish and aquatic systems: the climate challenge. Standing between life and extinction: ethics and ecology of conserving aquatic species in the American Southwest. D.L. Propst, J.E. Williams, K.R. Bestgen, and C.W. Hoagstrom, eds. Chicago, IL: University of Chicago Press. (invited, in preparation).
Parmesan, C. (2006). Ecological and evolutionary responses to recent climate change. Annual Review of Ecology, Evolution, and Systematics, 637-669.
Pörtner, H.-O., Karl, D. M., Boyd, P. W., Cheung, W. W. L., Lluch-Cota, S. E., Nojiri, Y., Schmidt, D. N., & Zavialov, P. O. (2014). Ocean systems. In C. B. Field, V. R. Barros, D. J. Dokken, K. J. Mach, M. D. Mastrandrea, T. E. Bilir, M. Chatterjee, K. L. Ebi, Y. O. Estrada, R. C. Genova, B. Girma, E. S. Kissel, A. N. Levy, S. MacCracken, P. R. Mastrandrea, & L. L. White (Eds.), Climate Change 2014: Impacts, Adaptation, and Vulnerability. Part A: Global and Sectoral Aspects. Contribution of Working Group II to the Fifth Assessment Report of the Intergovernmental Panel on Climate Change (pp. 411-484). United Kingdom and New York, NY, USA: Cambridge University Press.
Routson, C. C., Woodhouse, C. A., & Overpeck, J. T. (2011). Second century megadrought in the Rio Grande headwaters, Colorado: How unusual was medieval drought? Geophysical Research Letters, 38(22).
Udall, B., & Overpeck, J. (2017). The twenty-first century Colorado River hot drought and implications for the future. Water Resources Research, 53.
Van Mantgem, P. J., Stephenson, N. L., Byrne, J. C., Daniels, L. D., Franklin, J. F., Fulé, P. Z., Harmon, M. E., Larson, A. J., Smith, J. M., & Taylor, A. H. (2009). Widespread increase of tree mortality rates in the western United States. Science, 323(5913), 521-524.
Williams, A. P., Allen, C. D., Macalady, A. K., Griffin, D., Woodhouse, C. A., Meko, D. M., Swetnam, T. W., Rauscher, S. A., Seager, R., & Grissino-Mayer, H. D. (2013). Temperature as a potent driver of regional forest drought stress and tree mortality. Nature climate change, 3(3), 292-297.
Williams, A. P., Allen, C. D., Millar, C. I., Swetnam, T. W., Michaelsen, J., Still, C. J., & Leavitt, S. W. (2010). Forest responses to increasing aridity and warmth in the southwestern United States. Proceedings of the National Academy of Sciences, 107(50), 21289-21294.
Woodhouse, C. A., & Overpeck, J. T. (1998). 2000 years of drought variability in the central United States. Bulletin of the American Meteorological Society, 79(12), 2693.